INESC TEC Science Bits – Episode 3
PODCAST INESC TEC Science Bits (30:54 — 42,4 MB)
Guest Speakers:
Ariel Guerreiro, Centre for Applied Photonics (CAP)
Nuno Silva, Centre for Applied Photonics (CAP)
Keywords: Quantum Physics | Quantum Engineering | Quantum Technology | Quantum Sensors | Quantum Simulations | Photonics
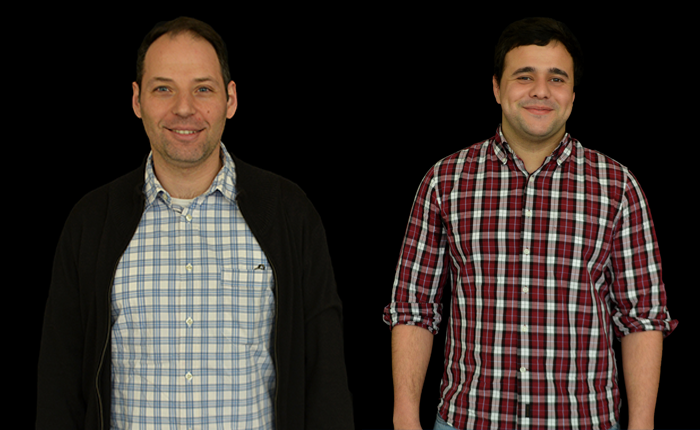
Is a second quantum revolution approaching?
The world is approaching a second quantum revolution that could transform industries, ranging from communications and Big Data to medicine and defence. This vision has encouraged large funding initiatives in Europe, the United States and China, which are prepared to spend billions of euros in research over the next years. The evolution of quantum science and technology is similar to the history of electricity: in the early eighteenth century, physicists like Volta, Ampère, Ohm and Maxwell established the foundations of electricity. Later, in the nineteenth century, names like Bell, Tesla, Edison and Hertz laid grounds for electrical engineering, by bringing electricity laws to the real world in the form of technology. As a result, the majority of electronic technology people use nowadays is based on the fundamental principles established by Faraday and his contemporaries. In this sense, we aim at doing exactly the same with quantum: to bring the principles and laws of quantum physics developed in the twentieth century by scientists like Planck, Einstein, Bohr and Schrödinger – who started the so-called “first quantum revolution” – into real world applications, and to inspire the second quantum revolution. As a result, a new type of engineering is evolving: Quantum Engineering.
What are quantum engineering and technology?
Quantum engineering is a multidisciplinary approach to quantum physics, focused on exploring quantum phenomenology to develop a novel technology, usually referred as quantum technology. But when does a technology become quantum? There is not a generalised agreement in the community on where the line between quantum and non-quantum technologies should stand. Those who advocate broader definitions argue that any technology supported by quantum features ought to be considered quantum. According to this definition, quantum technologies have been around for quite some time now, and we can consider modern electronics via the transistor and the laser as quantum technologies. However, stricter definitions require these technologies to explore more advanced quantum features.
Why are quantum laws different?
The differences between the macro and the microscopic world are not limited to the nature of the physical objects, and they have an impact on the notion of state of a system. Macroscopically, we think of objects as having well-defined properties: if a particle is here then it cannot be anywhere else; if a bit is zero, then it cannot be one. And if a cat is alive, then it cannot be dead. Well, in the quantum world, a particle can be in many places at once, a bit can be in a superposition of a zero and a one, and a cat can be both alive and dead (particularly cats of the Schrödinger variety). This ability constitutes the superposition principle of quantum physics, which is used to implement qubits, the quantum analogue of the bit used in quantum computers to store and manipulate information. In bounded systems such as atoms, quantum electronic states present a discrete spectrum of energy levels. This explains the spectroscopic response of atomic matter to light and provide the quantum elements necessary to produce lasers.
Why light?
Usually, the quantum behaviour of matter is only detectable in systems with scales very close to the size of the atom. On the other hand, various microscopic constituents (usually behaving incoherently) compose macroscopic systems; and although quantum effects dictate the inner mechanical gears of matter, they are usually masked by other phenomena. Thus, if we aim at developing quantum technologies, we must be able to manipulate and measure the behaviour of systems at very small scales or develop systems in which the various constituents interact coherently and cooperatively, in order to express their quantum behaviour at a macroscopic scale. Light is an essential tool to both approaches, because it is something that we can produce and manipulate on a macroscopic scale. On the one hand, it is a physical object with intrinsic quantum properties that can be used directly in a wide range of applications. On the other hand, it is used to control other quantum objects at the microscopic scale (e.g. individual atoms) or to promote specific interactions between multiple atoms, so they collectively express quantum effects at the macroscopic scale.
Centre for Applied Photonics (CAP): what do we do?
The development of quantum technologies and engineering at CAP began a few years ago; it aims at complementing the different, more historical research areas – each focused on exploring different fundamental properties of light in different applications. We’re talking about sensors using the wave properties of light, the manufacturing methods of integrated optics and microfluidics based on nonlinear optical processes, optical tweezers and traps resorting to the mechanical forces produced by light on small objects, etc. In this sense, there is a certain correlation between the different degrees of freedom of light and the different applications developed. Now, we intend to capitalise on the CAP’s experimental and technological expertise in applied photonics, in order to explore the potential of the quantum properties of light and matter, and to develop new technologies. To do so, we developed a plan focused on exploring systems with increasingly complex and complete degrees of quantum phenomenology involving light, starting from a semi-classical perspective that combines quantum and non-quantum elements, and evolving into a fully quantum framework.
Quantum sensing: why and how?
Sensing is expected to be the first real commercial success of quantum technologies, because it benefits from the extraordinary sensibility of quantum states to the environment. This feature is actually the main challenge in building large quantum computers, but for sensing, it acts as an enabler. Today, the market for quantum sensing already exceeds one hundred million euros and it is estimated to grow above 10% per year until 2024. These technologies range from atomic clocks and magnetic sensors to photonic quantum sensors and gravity sensors with applications covering sectors such as military and defence, automotive, healthcare, agriculture, oil and gas, and others.
The GreenNanoSensing project was born from this effort and it focuses on the development of optical functional metamaterials for sensing applications in green energies’ technologies, such as hydrogen detection or the measurement of electromagnetic fields. For example, we have developed a material that changes from transparent to opaque in the presence of small concentrations of hydrogen. In another line of research, we have designed new fibre optic sensors incorporating metallic nanostructures that try to access directly the quantum properties of plasmons (the quantum of excitation of the free charge density in conductive media) at the nanoscale. Hence, it is possible to develop optical systems that behave like artificial atoms with customised electronic and optical properties.
Quantum simulations: why and how?
So far, we have talked about how quantum laws are dramatically different from what we see in the macroscopic world. We now know the math behind it – Quantum Mechanics – and we are able to deal with it and to create new technology. For example, one can think about multiple ways to explore quantum laws to design new electronic or optical materials based on these properties at the microscopic level. But the main problem is trying to simulate such materials, which requires the simulation of the behaviour of a prohibitive number of degrees of freedom, i.e. equations, which is impossible, even with the best supercomputers we have available. In the 60s, Feynman suggested a way to work around this problem, arguing that if you were able to design a physical system following the same physical laws as the one you’re trying to simulate – call it an analogue system – then such system would act exactly as a computer, or an analogue quantum computer. This is like a “first generation quantum computer”, which we now call a quantum simulator or a simulator of quantum systems. The trick here is that, while the system we’re trying to simulate is inaccessible, the emulator – or quantum simulator – is at the macroscopic scale and can easily be controlled at the laboratory. Therefore, the current challenge is seeking analogue systems that we can work with, like versatile quantum simulation platforms through which we simulate a desired quantum system. Again, light can be instrumental here.